Design, Build & Test
What, how and why?
Modern engineering systems are becoming increasingly complex and interconnected, which requires a holistic approach, starting from conceptual design through mission design, operations analysis, and sustainment.
Multiphysics Multiscale Modeling, then, has become an important design tool for the development of new materials and components in severe environments. Unifying comprehensive 3D Multiphysics models of intricate geometries with 3D simulations of fluid flow, heat transfer in fluids and solids, and structural mechanics, should yield near realistic performance assessments of newly designed engineering components.
LIDAR-Drone Mapping for Mars Entry, Descent and Landing
As part of my research at the Mars Desert Research Station (MDRS), I led the development and test of an innovative drone-based LIDAR system aimed at enhancing Entry, Descent, and Landing (EDL) procedures in planetary exploration. Traditional EDL protocols often rely on aerial or orbital observations, which provide limited resolution for landing site analysis. My project sought to improve upon this by using a drone equipped with a LIDAR scanner, GPS, and an Inertial Measurement Unit (IMU) to generate high-resolution terrain maps, enabling more precise landing site selection.
Hardware Integration
The first step in this project was designing a custom 3D-printed mount for the LIDAR system. Using SolidWorks, my team and I created a lightweight yet durable mount tailored specifically for the DJI Mavic 2 Pro. The mount had to balance structural integrity with minimal weight to avoid exceeding the drone's payload capacity.
The LIDAR module was configured from scratch to ensure optimal data capture. This involved calibrating sensor parameters, adjusting scan frequencies, and implementing custom-made codes to obtain accurate point cloud mappings. Proper alignment of the LIDAR with the drone's center of gravity was also a critical consideration.
To power the LIDAR and auxiliary systems, my team and I carefully integrated LiPo batteries and voltage converters, ensuring a stable power supply without exceeding the drone's 700g maximum payload limit. This was determined through iterative testing to find the optimal weight-power tradeoff.
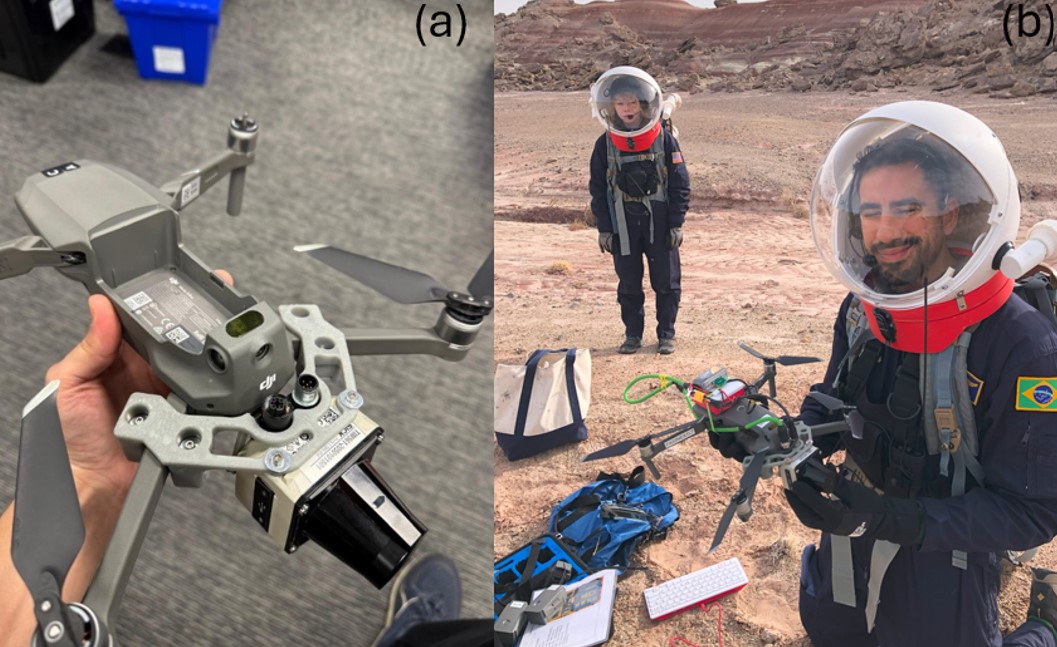
(a) Drone assembly with LIDAR sensor and mount; (b) Drone full assembly during an EVA with battery and onboard computing via Raspberry Pi.
Raspberry Pi & ROS2 Configuration
A Raspberry Pi was configured from scratch to serve as the central processing unit for data acquisition. I set up ROS2 (Robot Operating System 2) to handle real-time data streaming from the LIDAR, IMU, and GPS. The system was programmed to synchronize all data sources, enabling accurate mapping.
At MDRS, my team and I developed a custom SSH protocol to allow remote access to the Raspberry Pi during extravehicular activities (EVAs). This enabled real-time monitoring and debugging without direct physical interaction. Additionally, Python scripts were written to automate data collection, perform preliminary filtering, and facilitate post-processing of the LIDAR data to generate 3D terrain maps.
Tests
Throughout the mission, the drone system underwent iterative refinements based on field testing conducted during multiple EVAs. Early flights revealed mechanical instabilities in the LIDAR mount and electromagnetic interference affecting sensor readings. To address these issues, I reinforced the backup 3D-printed mount for safety and implemented additional radiation shielding for the cables. The Raspberry Pi onboard computer played a key role in managing power distribution and data acquisition, running custom scripts for automated logging and remote SSH control.
Further tests conducted at various MDRS locations demonstrated the enhanced capabilities of the improved system. The combination of LIDAR data, IMU gyroscopic readings, and GPS coordinates allowed the generation of detailed 3D point clouds of the terrain. These high-resolution maps provide valuable insights into potential landing hazards and safe zones, paving the way for future autonomous drone scouts on Mars.
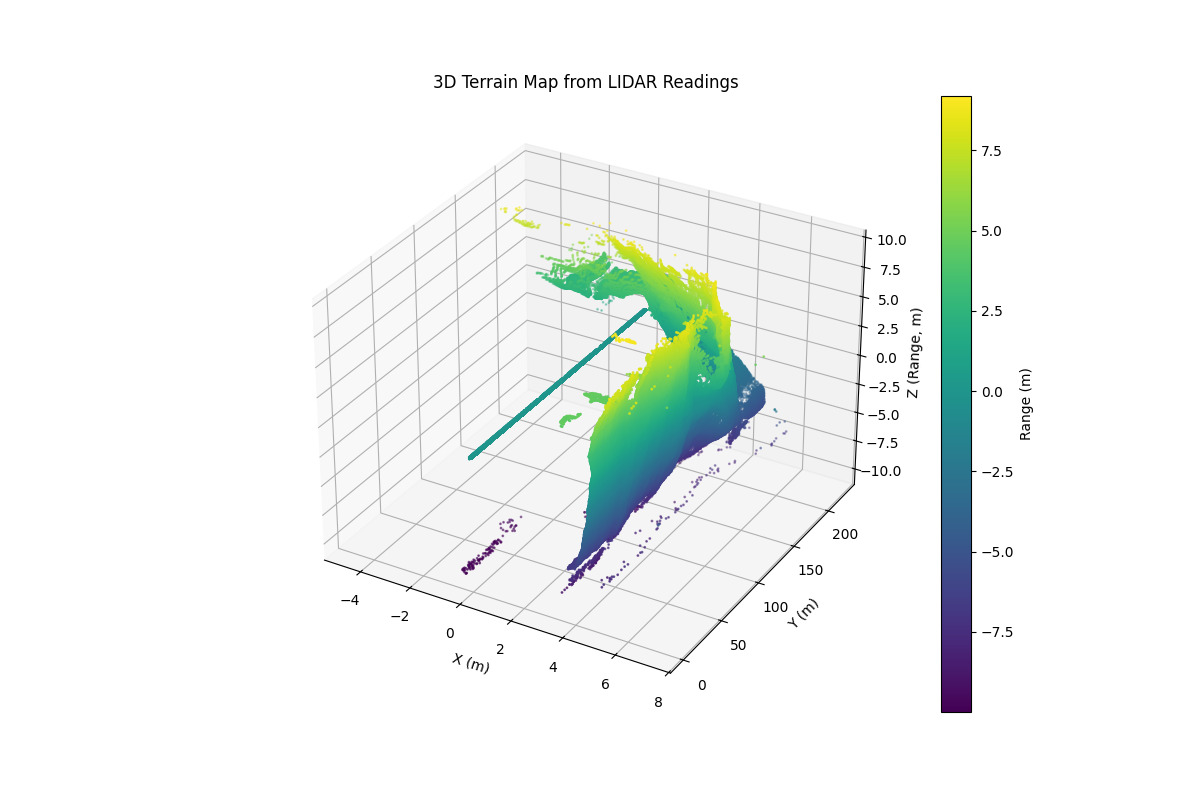
Point-cloud mapping of the LIDAR scan using the Raspberry Pi onboard computer.
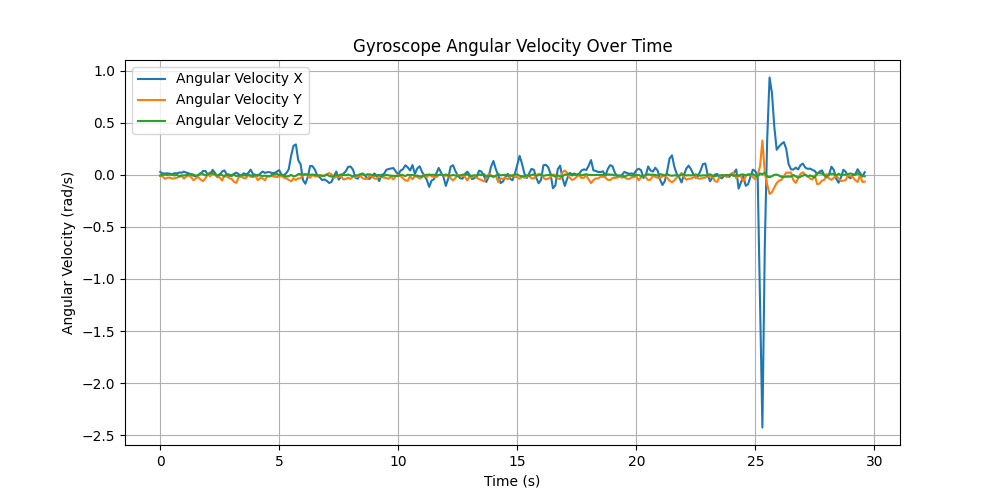
Gyroscope angular velocity readings during the mission.
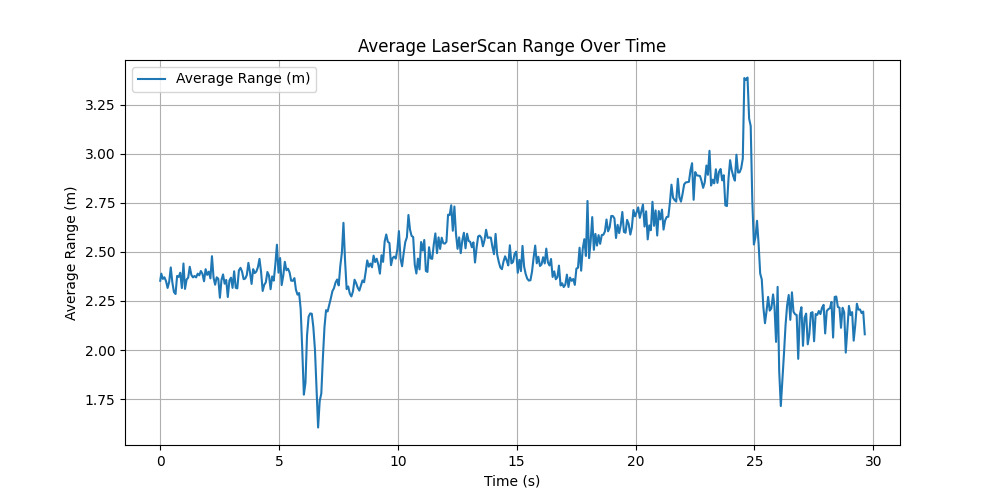
Mean value ranges of the LIDAR scans.
ACECam - Autonomous Computer Vision Embedded Camera
As the Chief Communications Officer of Translunar, I am actively involved in the development of ACECam, a cutting-edge optical navigation system designed for spacecraft engaged in relative navigation and proximity operations. ACECam is a compact, embedded vision system leveraging advanced image processing algorithms and AI-driven analytics to determine the precise position and orientation of spacecraft in orbit.
My contributions to this project focus on hardware integration, including assembling the system’s Raspberry Pi-based processing unit and calibrating the onboard camera for real-time image acquisition. The goal of ACECam is to enhance autonomy in space missions by enabling precise pose estimation and automated spacecraft docking. This technology is crucial for upcoming satellite servicing missions, constellation coordination, and in-space inspections.
With support from an Air Force STTR Phase I award, ACECam is currently undergoing rigorous testing to validate its capabilities. Future iterations will incorporate more robust AI models for improved object recognition and tracking, ensuring its effectiveness for commercial and military space applications.
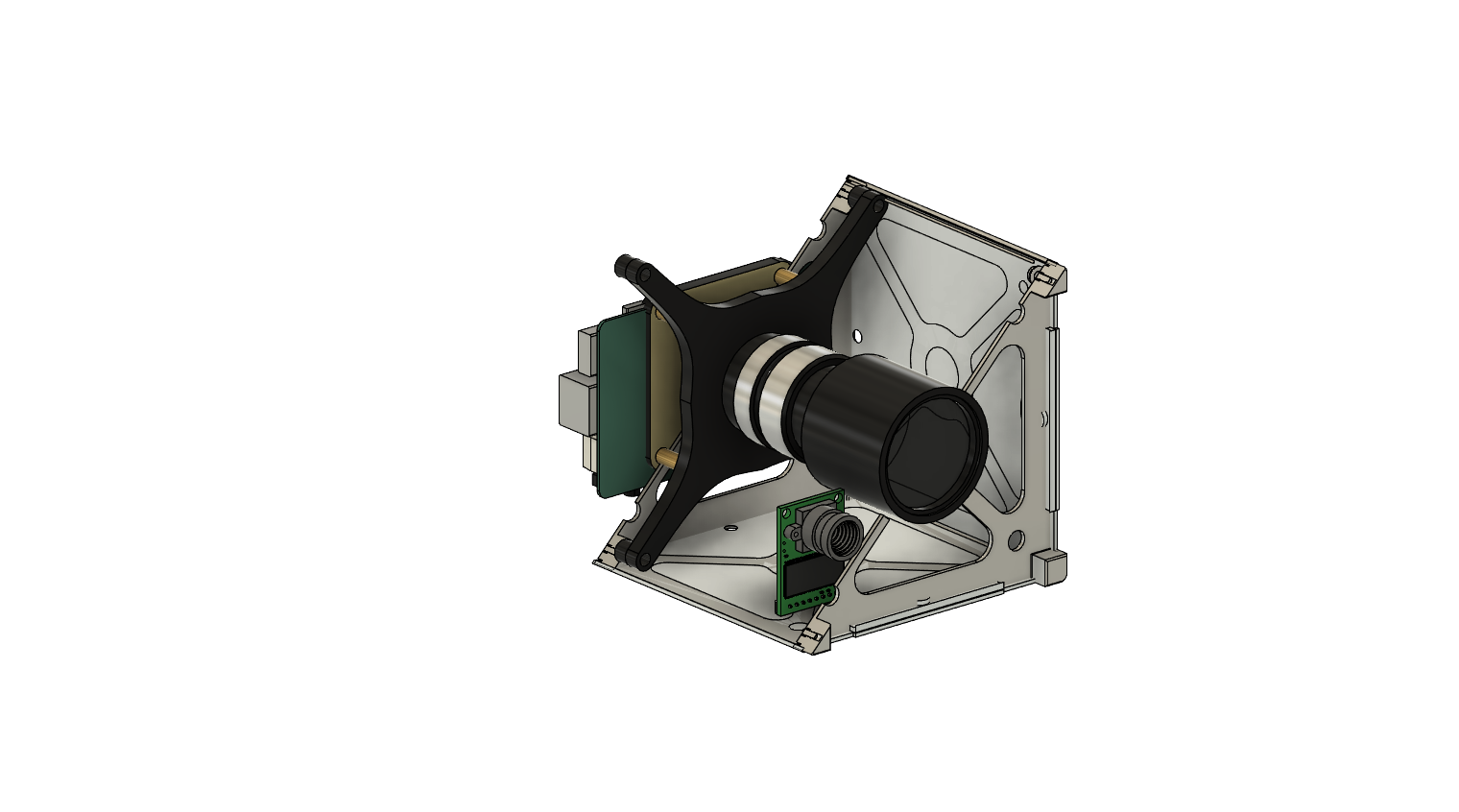
ACECam subsystem designed for optical navigation and proximity operations.
Rocketry
I worked for 1 year as an Aerodynamics & Structures member in University of Sao Paulo's rocket team, Project Jupiter. After that, I spent a semester as a Structures member in University of Notre Dame's Rocket Team, and came back as the Coordinator of Structures for another year in Project Jupiter, having the opportunity of working in four different rockets in total.
Preliminary Design
The first step for designing anything is the definition of the requirements. This involves iterations over the design, of course, but most importantly, it requires a structured approach to connect all the systems involved.
I implemented a simple method of optimizing the dimensions of our rockets, such as tail radius, nosecone length and fin parameters, through a merit function that is maximized when a) the static margin (distance from Center of Mass to Center of Pressure) is close to the optimal value of 2.0 and b) the area of the rocket fins is minimal, in order to diminish drag.
This analysis was implemented in our in-house 6 degree-of-freedom rocket trajectory simulator, RocketPy, which also makes use of the aerodynamic equations to foresee many characteristics of the rocket path.
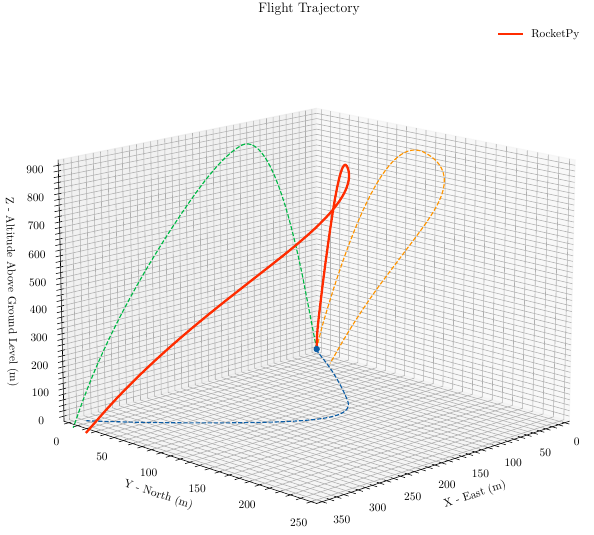
Rocket trajectory prediction using RocketPy (Project Jupiter's rocket trajectory simulator).
Computer Aided Design
Parallel to preliminary design, it is also necessary to iterate over the project's CAD. After working extensively with extruding, inspecting, defining joints, etc in Fusion 360, I've grown very fond of creating computer-aided designs.
CAD drawings for the rocket Imperius.
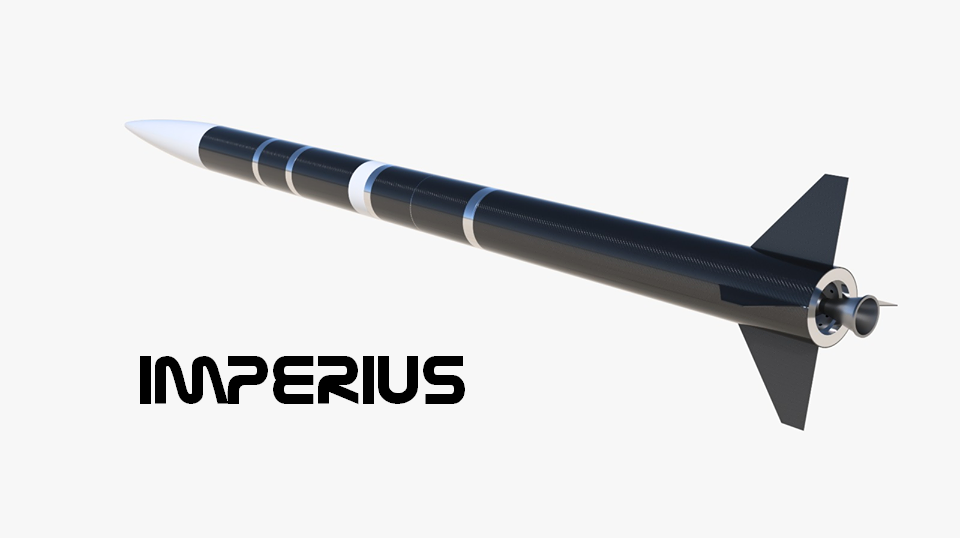
CAD 3D rendering for the rocket Imperius.
Simulation
Solid mechanics and fluid mechanics are an essencial part of simulating an engineered design before actually manufacturing it. Here, I worked mainly with structural analysis of joints using ANSYS Mechanical, even though I have also worked in CFD simulations in ANSYS Fluent.
Exploiting symmetry and defining forces for the structural analysis.
(Left) Von Mises Stress; (Right) Equivalent Strain.
Manufacturing
The final step in the design process is Manufacturing. I've had the opportunity of developing a vacuum infusion, carbon fiber laminated structure for three of Project Jupiter's rockets, through a technique that uses vacuum pressure to drive resin into a laminate. Materials are laid dry into the mold and the vacuum is applied before resin is introduced. Vacuum bagging greatly improves the fiber-to-resin ratio, and results in a stronger and lighter product.
Vacuum infusion process: Epoxy resin and hardener mixing; Vacuum infusion; Unmold; Final touch of resin.
This process was developed by us and used to build our rockets' tubes and fins, using a carbon/kevlar reinforced hybrid composite for the fins. We also laminated the structure externally for our rocket Callisto, whose launched can be seen here. Last but not least, for a few of our rockets we also 3D printed nosecones, using glass fiber reinforcement in the inside to make it more rigid.
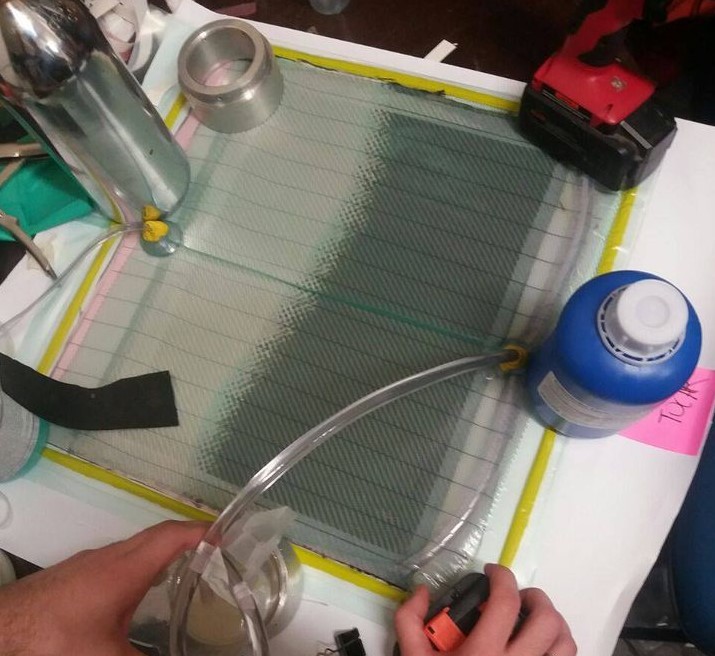
Fin vacuum infusion lamination: the dark part is resin spreading on the carbon fiber piece with the assistance of a vacuum pump, which prevents undesired bubbles.
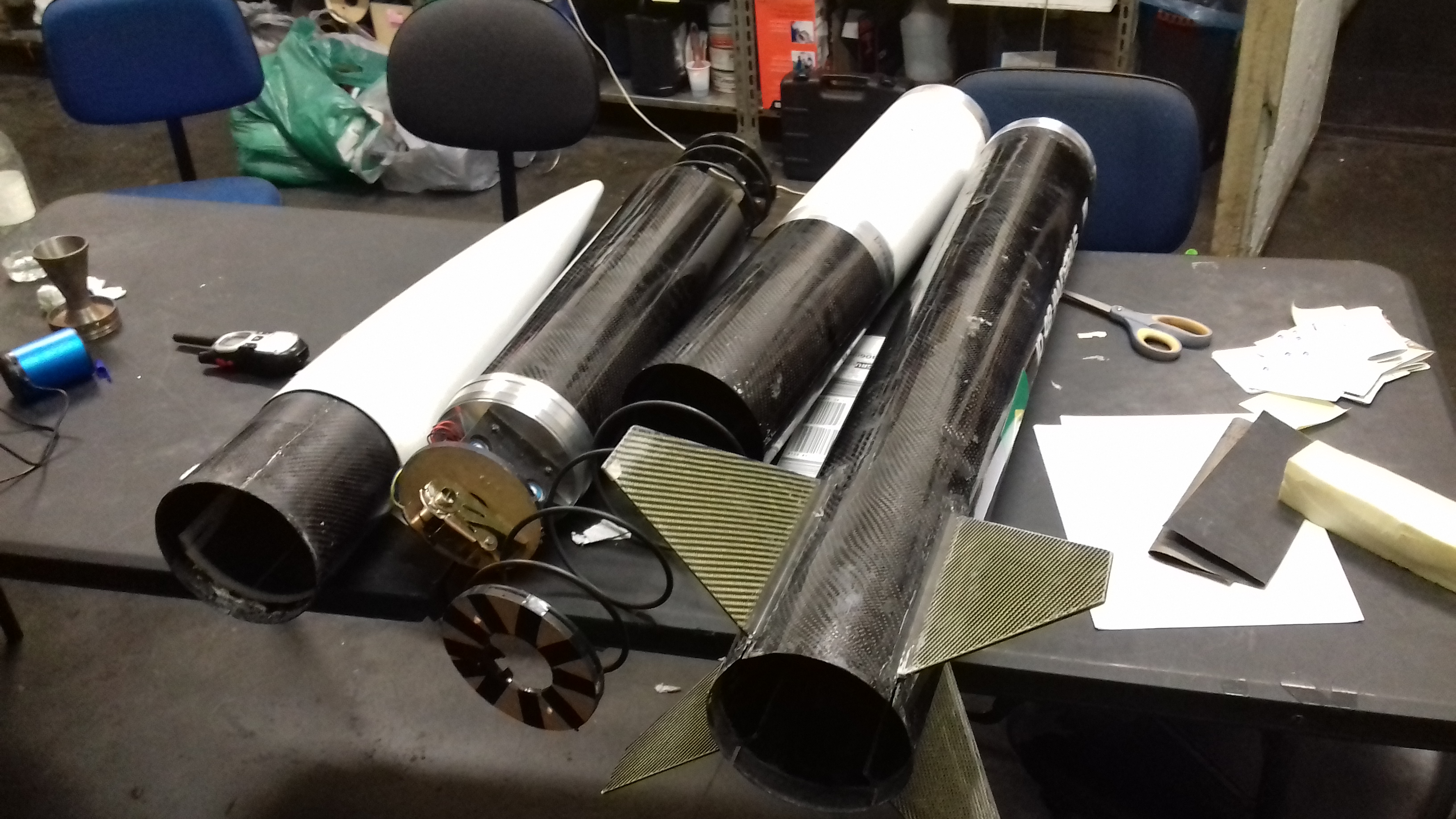
Final carbon fiber structure for the rocket Imperius: 5 carbon fiber tubes, glass fiber reinforced nosecone and 4 carbon fiber/kevlar fins.
Academic Background
Introduction to Mechanical Engineering Design and Manufacturing with Fusion 360
AAE 539 - Advanced Rocket Propulsion
AME 20241 - Solid Mechanics
4300324 - Fluid Mechanics
4302305 - Mechanics I
4300463 - Applied Physics
4302401 - Statistical Mechanics
4300402 - Introduction to Solid State Physics
4302113 - Experimental Physics I
4302114 - Experimental Physics II
4302213 - Experimental Physics III
4302214 - Experimental Physics IV
4302313 - Experimental Physics V
Autodesk (on Coursera) (~12 hours)
The manufacturing industry is making a digital transformation, allowing companies to customize production through advances in machine learning, sustainable design, generative design, and collaboration, with integrated design and manufacturing processes. This course introduces innovations in CAD and digital manufacturing, speaking to the rapid changes taking place that are forever transforming the future of making. This course will also explore foundational concepts behind Autodesk® Fusion 360™ CAD/CAM. Fusion 360 is a cloud-based CAD/CAM tool for collaborative product development that combines industrial design, mechanical engineering, and machine tool programming into one software solution. Through a series of lectures and hands-on exercises, this course provides the core philosophy behind the software. By understanding how designs are both made and assembled, you'll learn to create better designs from the start.
Autodesk (on Coursera) (~17 hours)
This course explores the design for manufacture workflow and shows how to validate models and create the G code, the programming language needed to instruct the CNC machine on how to move. We practice the basics of part and assembly design, and tools such as animation, rendering, and simulations using Autodesk Fusion 360. We learn the basics in each of these areas which are to be fully developed in later courses that apply these principles and Fusion 360 skills. After taking this course, you'll be able to: Explain the design to manufacturing process used to take a digital model to a physical part through CNC programming; Summarize the toolset available in Fusion 360; Demonstrate knowledge and skills in Fusion 360 applying design and manufacturing workflows to take digital parts to physical prototypes.
Prof. Tim Pourpoint - Purdue University
AAE 53900, Advanced Rocket Propulsion, presents a graduate-level treatment of topics related to chemical rocket propulsion. Following a brief review of rocket fundamentals, the course provides a detailed discussion on thermochemistry and chemical equilibrium relating these concepts to the structure and operation of standard industry codes like the NASA Chemical Equilibrium with Applications (CEA) code. The next section of the course provides fundamentals of incompressible and compressible flows as applied to key elements of chemical propulsion systems such as propellant feed systems and nozzles. Before providing an in-depth look at solid, liquid, and hybrid propulsion systems, the course provides a review of fundamental heat transfer processes as applied to chemical rockets. The solid and hybrid rocket sections of the course include a review of ballistic models, burning rate theory, and erosive burning among other topics. The liquid rocket section of the course includes discussions on engine cycle analysis and turbopump design. In a typical semester, the course involves about seven homework assignments (including two in-depth homework assignments requiring more analyses and time than a traditional homework), and two exams (a midterm and a final).
Topics Covered:
Generalized internal compressible and incompressible flows. Thermochemistry and heat transfer in rocket applications. Advanced topics in solid rocket motor performance and internal ballistics, hybrid rockets, and thermal-nuclear engines. Liquid rocket engine cycle analysis and turbopump design.
Prof. Michael Sangid - Purdue University
This course serves as an overview for materials behavior for students without a materials background, including seniors and entry-level graduate students. Materials are at the foundation for all of engineering, as evident by the latest products that we design, to the airplanes that we fly, to the latest smart phones. In fact breakthroughs with material research are often accompanied by rapid advancements in technology. Thus it is paramount for all engineers to have an understanding of the structure and behavior of materials.
In this class, we focus on the structure of materials, the microstructure connection to mechanical properties, and ultimately failure mechanisms. Materials play an important role in both design and manufacturing, which will be addressed in the context of components and extreme environments. Of specific interest will be defects within materials, defect formation/evolution, and their role in strengthening mechanisms.
Material anisotropy, micromechanisms, and elasto-plastic properties at the atomic, single-crystal/ constituent, and polycrystal/material levels and their use in explaining the deformation and failure characteristics in metals, polymers, and ceramics; failure mechanisms and toughening in composites; structure and behavior of aerospace materials: metal alloys, ceramic-matrix composites, and fiber-reinforced polymer composites. Particular topics will also include: elastic deformation, dislocation mechanics, plastic deformation and strengthening mechanisms, creep, and failure mechanisms; design criteria; special topics. We will attempt to have minimal overlap with AAE 554 Fatigue of Structures and Materials, therefore we will not cover fracture, fatigue, or stress concentrators.
Topics Covered:
- Indicial Notation
- Crystallography
- Elasticity
- Stress-Strain Relationships and Yielding
- Dislocation Mechanics
- Partial Dislocations and Stacking Faults
- Crystallographic Slip
- Twinning and Shape Memory Effects
- Strengthening Mechanisms
- Creep
- Two Bar Problems and Residual Stress
- Ceramics, Probability of Failure, and Statistical Variations
- Polymer Structure, Deformation, Fracture, and Viscoelasticity
- Composites
Prof. Pinar Zorlutuna - University of Notre Dame (45 hours)
Stresses, strains, loads, deformations and displacements in tension, compression, shear, torsion and bending;
Elasticity and inelasticity;
Strain energy;
Transformations of stress and strain;
Buckling;
Combined loadings;
Thermal effects.
Prof. Frederique Grassi - University of São Paulo (60 hours)
1. Notion of fluid.
2. Eulerian and Lagrangian descriptions.
3. Conservation laws, Euler equation (perfect fluid).
4. Statics.
5. Stationary flows.
6. Vorticity and rotational flow.
7. Potential movement.
8. Viscosity, Navier-Stokes equation,
9. Similarity, Reynolds number, Stokes equation, boundary layer.
10. Waves: surface, sound, etc.
11. Instability, intermittency, turbulence.
Prof. Airton Deppman - University of São Paulo (60 hours)
1. Newton's Laws: Movement of a particle in one dimension, conservative forces.
2. Movement of a particle in two or three dimensions, angular momentum, central forces.
3. Theory of small oscillations, normal modes of vibration and normal coordinates. Coupled Oscillators.
4. Coordinate systems in motion: Non-inertial systems.
5. Variational calculation. Lagrange equation and Hamilton principle.
Prof. Márcia Fantini - University of São Paulo (60 hours)
History of science with emphasis on the technical and science relationship. Materials Science: metals, insulators, semiconductors, superconductors, magnets. Energy: classic and alternative sources. Operation and concepts related to everyday devices: engines, sound, images, etc. Human sensitivity: eye, ear, nerves. Laser and holography.
Prof. Mario de Oliveira - University of São Paulo (60 hours)
1. The laws of thermodynamics.
2. Notions of probability.
3. Microchanonic representation. Boltzmann's entropy.
4. Canonical representation. Maxwell distribution of speeds. Partition function and connection with thermodynamics.
5. Einstein model for the specific heat of solids. Ideal monoatomic gas. Gibbs' paradox. Ideal diatomic gas.
6. Photon gas, thermal radiation. Phon gas, linear chain, Debye theory.
7. Grand canonical representation. Distribution of Bose-Einstein and Fermi- Dirac.
8. Free electron gas, electronic thermal capacity.
9. Gases and liquids, integral configuration, according to virial coefficient, van de Waals theory.
Prof. Lucy Assali - University of São Paulo (60 hours)
Crystalline structure. X-ray diffraction and reciprocal network. Crystalline bonds. Network vibrations, phonons and thermal properties. Free electron Fermi gas. Energy bands. Semiconductors. Metals and Fermi surfaces. Optical processes. Magnetism. Superconductivity.
Prof. Alexandre Correia - University of São Paulo (60 hours)
To explore experimental aspects of physics with regard mainly to concepts of mechanics through:
1. Simple measurements of dimensions, time and mass and derived quantities
2. Studies of motion kinematics, measurements of speeds and accelerations.
3. Dynamics of movement: study of force interacting over bodies
4. Laws of conservation of energy and momentum: collisions, conversion of potential kinetic energy and vice versa
Introduce the bases of modern scientific Projects through:
1. Discussion of the scientific method
2. Notions of ethics in science
3. Safety in the laboratory.
Through performing simple execution experiments, develop skills to:
1. practice careful and systematic data collection;
2. identify the existence and quantify experimental uncertainties;
3. develop a critical analysis of the data set.
For data processing, formally introduce the concepts of:
1. direct and indirect measures;
2. notions of instrumental uncertainties and their effect on the conclusion of an analysis.
3. Notion of precision.
4. notions of simple graphic analysis. Linear, monolog and dilog scales. Visual extraction of line coefficients.
5. basic notions of statistics: mean, standard deviation, standard deviation of the mean.
For the analysis, synthesis and presentation of the results:
1. systematize the presentation and analysis of data through tables, graphs and histograms;
2. introduce computational tools for data processing, graphics and text writing;
3. criticize discrepancies found, taking into account experimental and theoretical limitations involved in obtaining the results;
4. elaborate the synthesis of the experiment, properly selecting the information obtained.
Prof. Alexandre Correia - University of São Paulo (60 hours)
To study physical phenomena involving mainly aspects of mechanics and thermodynamics, such as:
1. Uniform and accelerated movements in viscous media
2. Conservation laws in mechanics
3. Kinematics and dynamics of rigid bodies
4. Oscillations
5. Heat physics, temperature measurements , specific heat, phase transitions
Improve the foundations of modern scientific Projects through:
1. Discussion of the scientific method
2. Notions of ethics in science
3. Safety in the laboratory.
Through performing simple execution experiments, develop skills to:
1. practice careful and systematic data collection;
2. identify the existence and quantify experimental uncertainties;
3. develop a critical analysis of the data set.
For data processing, formally introduce the concepts of:
1. direct and indirect measures;
2. propagation of uncertainties;
3. Gaussian distributions;
4. least squares method. Adjustments of linear functions.
5. Significance tests focusing on the z-test.
For the analysis, synthesis and presentation of the results:
1. systematize the presentation and analysis of the data through graphic tables and histograms;
2. emphasize the use of the computer for organizing and analyzing data. It is possible to expand the use of the computer, stimulating the use of simulations in the description and forecast of the results;
3. Obtain from the data compilation the information about the experiment and the physical phenomenon in question;
4. perform comparisons of results obtained by different methodologies;
5. criticize discrepancies found, taking into account experimental and theoretical limitations involved in obtaining the results;
6. prepare the synthesis of the experiment, properly selecting the information obtained.
Prof. Alexandre Suaide - University of São Paulo (120 hours)
Experimentally study aspects of electromagnetism, among which:
1. Electric circuits in direct and alternating current
2. Measurements of electric and magnetic fields
3. Movement of particles in electric and magnetic fields
4. Aspects of the laws of Gauss, Ampère and Faraday
Through the carrying out diverse experiments, developing skills to:
1. practice careful and systematic data collection;
2. identify the existence and quantify experimental uncertainties;
3. develop a critical analysis of the data set.
For data processing, formally introduce the concepts of:
1. probability distributions, including Gaussian, binomial and Poisson;
4. Various methods of adjusting functions. Adjustments of non-linear functions.
5. Tests of significance. Student's t-test
For the analysis, synthesis and presentation of results:
1. systematize the presentation and analysis of data through graphical tables and histograms;
2. continue to encourage the use of computers for organizing and analyzing data. It is possible to expand the use of the computer, stimulating the use of simulations in the description and forecast of the results;
3. Obtain from the data compilation the information about the experiment and the physical phenomenon in question;
4. perform comparisons of results obtained by different methodologies;
5. criticize discrepancies found, taking into account experimental and theoretical limitations involved in obtaining the results.
Prof. Valmir Chitta - University of São Paulo (120 hours)
Experimentally study aspects of electromagnetism, including:
1. Alternating current electrical circuits
2. Electromagnetic fields
3. Light wave properties: reflection, refraction, interference and diffraction
4. Spectrophotometry at frequencies close to that of visible light
Through the realization of experiments that require the systematic performance of experimental measures and their correlations:
1. practice careful and systematic data collection;
2. automate experiments;
3. correlate independent data sets in order to extract a more complex physical interpretation;
4. develop a critical analysis of the data set.
For data processing, formally introduce the concepts of:
1. experimental simulations, Monte Carlo method;
2. adjustments of generic and non-linear functions. Maximum likelihood method;
3. analysis of correlated data (covariance);
4. propagation of uncertainties with covariance between parameters;
5. extrapolation of curves;
6. handling of large volumes of data;
7. systematic measurement uncertainties.
For the analysis, synthesis and presentation of results:
1. elaborate syntheses of experiments, properly selecting the information obtained and correlating them with previously performed measures;
2. develop oral presentations of experimental results.
Prof. Rosangela Itri - University of São Paulo (120 hours)
Experiences that supported the formulation of Quantum Mechanics.
Through the performance of complex experiments, which require the systematic performance of experimental measures and their correlations:
1. practice careful and systematic data taking;
2. automate experiments;
3. correlate independent data sets in order to extract a more complex physical interpretation;
4. develop a critical analysis of the data set.
For data processing, perfect the concepts of:
1. experimental simulations, Monte Carlo method;
2. adjustments of generic and non-linear functions. Maximum likelihood method;
3. analysis of correlated data (covariance);
4. propagation of uncertainties with covariance between parameters;
5. extrapolation of curves;
6. handling of large volumes of data;
7. systematic measurement uncertainties.
For the analysis, synthesis and presentation of results:
1. elaborate syntheses of experiments, properly selecting the information obtained and correlating them with previously performed measures;
2. develop oral presentations of experimental results.